Update(MM/DD/YYYY):05/20/2016
Development of an 11 % Conversion Efficiency Thermoelectric Module
- Achieved with thermoelectric materials having controlled nano-sized structures, a big step toward the realization of power generation using waste heat –
Points
-
Made dramatic improvements in the performance by forming nanostructures in the sintered compacts of lead telluride (PbTe)-based thermoelectric materials
-
Developed a thermoelectric module using PbTe sintered compacts with nanostructures, achieving conversion efficiency of 11 %
-
Made a big step toward power generation utilizing waste heat which reaches up to 60 % of primary energy
Summary
Michihiro Ohta (Senior Researcher), Atsushi Yamamoto (Leader), Xiaokai Hu (former AIST Postdoctoral Researcher), and Priyanka Jood (JSPS Postdoctoral Fellow), the Thermoelectric Energy Conversion Group, the Research Institute for Energy Conservation (Director: Tetsuo Munakata), the National Institute of Advanced Industrial Science and Technology (AIST; President: Ryoji Chubachi), have achieved a high thermoelectric figure of merit ZT = 1.8 by forming magnesium telluride (MgTe) nanostructures in a sintered compact of lead telluride (PbTe)-based thermoelectric material, and have used this material to develop a thermoelectric module with conversion efficiency of 11%.
Until now, it has been difficult to exceed ZT = 1.0 in thermoelectric materials, or 7 % conversion efficiency in thermoelectric modules. In the present research, in collaboration with Mercouri G. Kanatzidis (Professor of Northwestern University and also Senior Researcher at the Argonne National Laboratory in the U.S.), the researchers have enhanced the ZT of a sintered compact of thermoelectric material to 1.8 (550 °C) using a nanostructure forming technology. Furthermore, they have developed an electrode material that contacts relatively well electrically and thermally with PbTe sintered compact containing the MgTe nanostructures, achieving a conversion efficiency of 11 % (hot side: 600 °C, cool side: 10 °C) in the thermoelectric module. This research has paved the path to converting waste heat to useful electrical power on large-scale practical applications .
The details of this technology will soon be published in Energy & Environmental Science, an academic journal published by the Royal Society of Chemistry of the U.K.
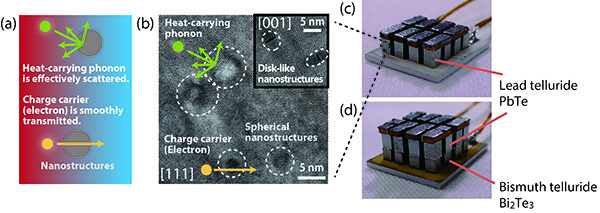 |
Figure 1: Conceptual diagram of improvement in thermoelectric figure of merit ZT (a), transmission electron micrograph of the developed thermoelectric material (b), and photographs of the thermoelectric modules (c) (d)
(a) (b) Because the nanostructures formed in the PbTe sintered compact effectively scatter heat-carrying phonons, while not having effect on the charge carrier transport, it dramatically improves the ZT of PbTe. (c) One-stage and (d) two-stage (segmented) thermoelectric modules using the PbTe sintered compacts with the nanostructures.
|
Social Background of Research
Japan relies on imports for most of its primary energy (such as oil and coal), but over 60 % of that is simply discarded as unused waste heat. In order to achieve a stable supply of energy and a sustainable society, there is a need to promote the use of this waste heat. The key to this lies in the technology of thermoelectric power generation which allows the direct conversion of “mottainai” waste heat into electrical energy.
Until now, it has been difficult to realize thermoelectric materials that exceed ZT = 1.0, but in recent years, the development of thin films and melt grown ingots of thermoelectric materials exceeding ZT = 1.0 have been made possible through the use of nanotechnology. However, this progress in the materials field could not be applied to thermoelectric modules, so commonly obtainable one-stage thermoelectric modules remained at conversion efficiency of 5 % or less, and two-stage (segmented) thermoelectric modules being developed at the laboratory level remained at conversion efficiency of about 7 %. In order to apply progress in the materials field to thermoelectric modules, it is essential to improve ZT in sintered compacts suitable for processing.
History of Research
With the aim of utilizing waste heat through thermoelectric power generation, AIST has made efforts in research and development of a wide range of fields related to thermoelectric conversion technology from materials to modules, and their evaluation. There are only a few institutions in the world studying all aspects of thermoelectric technology.
Until now, it has been difficult to exceed ZT = 1.0 in thermoelectric materials, but since the 2000s, it has been demonstrated that by forming nanostructures in thermoelectric materials, it is possible to scatter phonons effectively and reduce thermal conductivity, improving ZT dramatically as a result. AIST has focused on PbTe-based thermoelectric materials that can be used in a temperature range of 300 °C to 700 °C, and in collaboration with Northwestern University and the Argonne National Laboratory, embedded MgTe nanostructures in p-type PbTe melt grown ingots, confirming improvement of ZT (ZT = 1.6) in 2012. In the present research, AIST developed a sintered compact of a thermoelectric material having a high ZT due to the formation of nanostructures. Furthermore, using AIST’s expertise on thermoelectric technology, an electrode material was developed that contacts relatively well electrically and thermally with the developed thermoelectric material with nanostructures, aiming for highly efficient thermoelectric modules.
This research and development was conducted with the support of the Ministry of Economy, Trade, and Industry’s commissioned projects of Japan-U.S. Cooperation Project for Research and Standardization of Clean Energy Technologies (FY2010 to FY2014).
Details of Research
In thermoelectric materials, in order to achieve high conversion efficiency from heat into electrical power, high electrical conductivity and low thermal conductivity are needed . In this research, AIST succeeded in embedding MgTe nanostructures in p-type PbTe sintered compacts (using sodium (Na) as the acceptor) which are favorable for processing into thermoelectric modules (Fig. 2 (a)). Formation of spherical nanostructures and plate-like nanostructures of about 5 nm can be observed in a transmission electron micrograph of the sintered compacts (Fig. 1 (b)). The embedded MgTe nanostructures effectively scatter phonons, reducing thermal conductivity (Fig. 1 (a)). Because PbTe and MgTe have a smooth interface, the band offset of PbTe and MgTe is very small, and it has only a small effect on the transmission of charge carriers between two components. As a result of reducing only the thermal conductivity without affecting the electrical properties, as shown in Fig. 2 (b), the ZT of this PbTe sintered compact with the MgTe nanostructures was improved dramatically to 1.8 at 550 °C (approximately two-fold compared to conventional PbTe).
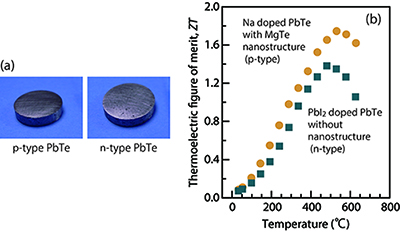 |
Figure 2: Exterior of the developed PbTe (p-type) with the MgTe nanostructures and the PbTe thermoelectric material used for n-type (a) and temperature dependence of ZT (b)
Sodium was used as the acceptor in the p-type and PbI2 was used as the donor in the n-type. |
Furthermore, with AIST’s expertise on thermoelectric conversion technology, AIST conducted the research and development of thermoelectric conversion modules (Fig. 1 (c)), using the p-type PbTe sintered compacts with the MgTe nanostructures and n-type PbTe sintered compacts (using lead iodide (PbI2) as a donor, exterior shown in Fig. 2 (a) and ZT in Fig. 2 (b)). In this work, an electrode material composed of iron (Fe) and cobalt (Co) was developed which was found to contact relatively well to the highly efficient thermoelectric conversion material (PbTe sintered compacts with MgTe nanostructures) both thermally and electrically. As shown in Table 1, this resulted in very high maximum power output (3.55 W) and maximum conversion efficiency (8.8%) for a one-stage module (hot side temperature 600 °C, cool side temperature 30 °C). Here, the size of one thermoelectric element was 2.0 mm long × 2.0 mm wide × 2.8 mm tall, and the developed thermoelectric module consisted of eight pn element pairs.
The PbTe sintered compacts with MgTe nanostructures exhibited high ZT in a temperature range from 300 °C to 700 °C, but low ZT below 300 °C (Fig. 2 (b)). Therefore, typical bismuth telluride (Bi2Te3) that exhibits high ZT (about 1.0) at 100 °C was used as the cool side element to develop a two-stage (segmented) thermoelectric module (Fig. 1 (d)). By improving the efficiency of the cool side, maximum power output reached 2.34 W and maximum conversion efficiency reached 11 % with hot side at 600 °C and cool side at 10 °C (Table 1). This thermoelectric module was composed of eight pn element pairs, and the PbTe thermoelectric element size was same as that of the one-stage type, with Bi2Te3 thermoelectric conversion element size of 2.0 mm long × 2.0 mm wide × 2.0 mm tall.
With the finite element method using commercially available software COMSOL Multiphysics ®, the performance of the thermoelectric modules was simulated from the thermoelectric properties of the PbTe sintered compacts with MgTe nanostructures (Fig. 3). As shown in Table 1, it was found that if an ideal thermoelectric module could be developed, maximum conversion efficiency of 12.2 % in a one-stage thermoelectric module and 15.6 % in a two-stage thermoelectric module could be achieved. After analysis of the simulation results, it was concluded that the difference between the measured values and the simulated values was due to the large electrical resistance at the interface between the PbTe sintered compacts and the electrode material, and due to the heat loss that does not contribute to power generation, such as radiation. In the future, if the electrical resistance at the interfaces and geometrical configuration of the module can be improved, it is possible to achieve efficiency significantly greater than 11 %.
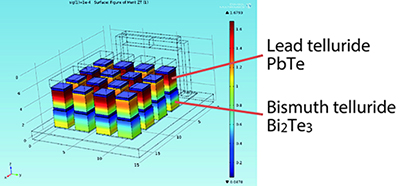 |
Figure 3: Simulation using the finite element method
This shows the distribution of ZT of the two-stage thermoelectric module. |
Future Plans
AIST will work to improve electrical and thermal contacts between thermoelectric materials with nanostructures and electrode materials, and achieve further improvement in power output and conversion efficiency. In addition, AIST will conduct research and development related to improving long-term durability and mechanical properties of the developed thermoelectric modules, and conduct experiments with industry aiming at practical use within 5 years. Simultaneously, the researchers will seek element substitution, such as replacing the toxic element lead (Pb) with less toxic element copper (Cu), and replacing the rare element tellurium (Te) with earth-aboundant element sulfur (S). AIST will advance research and development based on the present results, opening the path to innovative conversion of electric power from waste heat, and contribute to a sustainable society.