- Prospective applications include a two-color light source of infrared light and X-ray with variable energy -
Kawakatsu Yamada (Deputy Director of Research Institute of Instrumentation Frontier) and, Norihiro Sei (Senior Research Scientist) and Hiroshi Ogawa (Research Scientist) of the Quantum Radiation Research Group, the Research Institute of Instrumentation Frontier (Director: Yoshio Akimune) of the National Institute of Advanced Industrial Science and Technology (AIST) (President: Tamotsu Nomakuchi) have developed a compact free electron laser (FEL) that can simultaneously emit high-intensity short pulse beams of monochromatic infrared light and X-ray.
In this study, we used an electron storage ring NIJI-IV for the FEL that was jointly developed by AIST and Kawasaki Heavy Industries, Ltd. We additionally developed an optical klystron ETLOK-III, a light source of length 3.6 m with a long undulator period and strong magnetic field, and a highly stable optical resonator system with a vibration displacement of less than 0.5 µm. By combining them, an infrared FEL is developed with a maximum output of 1.6 mW and wavelength of 0.84–1.50 µm (infrared light region).
Quasi-monochromatic X-rays are successfully generated in the optical resonator in which an infrared FEL beam collides with a bunch of electrons moving at a speed close to the speed of light. The number of generated X-ray photons is roughly 106 photons/s with the energy (equivalent to wavelength) of 1.2–2.1 MeV. It is to be noted that the realized energy range overlaps the range of 0.3–1.5 MeV where a monochromatic X-ray source has so far been believed very difficult to realize.
A prospective application of the present system would be a high-flux two-color light source of infrared light and an X-ray with variable energy (equivalent to wavelength). If applied to the accelerator such as an energy-recovery linac, one would be able to realize a quasi-monochromatic X-ray source with the energy of over 0.3 MeV and the yield of 1012 photons/s, of the level not easily attainable even in a large synchrotron radiation facility. This type of X-ray beam would offer new measuring methods for magnetic material research, and would also contribute to the development of an ultra-high density magnetic recording system.
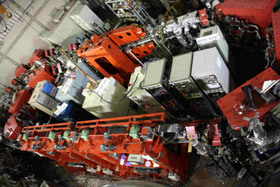 |
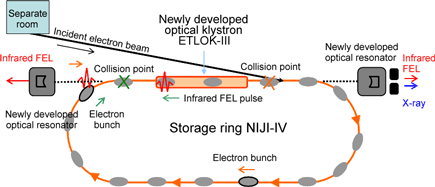 |
Picture (left) and schematic view (right) of the electron storage ring NIJI-IV for a FEL |
When an electron beam turns in some other direction at a speed close to the speed of light, the tangentially emitted light can be used as a highly directional continuous wavelength light source. The construction of electron storage rings commenced in the 70's, which aimed at realizing such light sources. To increase the photon energy, the size of the facility was continuously increased, and high-intensity light sources with the energy of up to several 10 keV have been developed; they contribute to material and biological sciences and the growth of industries. However, it is still difficult to obtain an X-ray beam with the energy of over 0.3 MeV.
The interaction between materials and X-rays in the energy range of 0.3–1.5 MeV can be effectively used for measuring the distribution of the kinetic momentum density of electrons as an indispensable means of studying the magnetic properties of alloys and thin films. It is widely believed that any new X-ray source, if developed, would surely pave the way for new applications.
AIST made an effort to shorten the wavelength of a FEL through the use of NIJI-IV, and achieved the shortest wavelength and developed the nation's first laser oscillation in the vacuum ultraviolet region. On the basis of the advanced FEL technology developed so far, a project was recently started to lengthen the wavelength of a storage ring FEL.
This study is performed in the research project "study on the broad-band quantum radiation source using a FEL and related advanced utilization technologies (2004-2008)," which is based on the evaluation by Atomic Energy Commission and funded by Ministry of Education, Culture, Sports, Science and Technology.
Within the energy range of 0.3–1.5 MeV, it is possible to obtain monochromatic high-energy X-rays by inverse Compton scattering, in which a laser beam collides with a bunch of electrons moving at a speed close to the speed of light. For using electron bunches in a storage ring, the electron energy must be relatively low and the laser wavelength must be in the infrared region. To obtain an X-ray beam by such means, AIST started to develop an infrared storage ring FEL system.
NIJI-IV has two long straight sections: one is already assigned for the development of short-wavelength FEL beams and the other one is used as an infrared beam line for developing an infrared storage ring FEL. An optical klystron ETLOK-III, as shown in Fig. 1, is fabricated and placed in the long straight section of NIJI-IV as an insertion device.
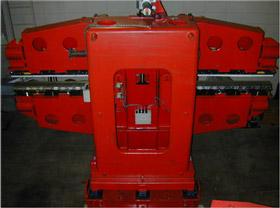 |
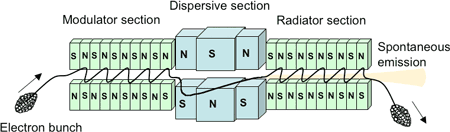 |
Fig. 1 Picture (left) and schematic view (right) of optical klystron ETLOK-III |
The optical klystron ETLOK-III is 3.6-m long and occupies roughly half the length of the straight section. Because of its compact size, we can place collision points for the inverse Compton scattering of FEL photons in the regions outside the magnetic field. Within a relatively small total length of the klystron, we place a 0.75-m dispersion section with strong magnetic field in the center, which is sandwiched by two 1.4-m undulators (the green portions in the schematic view of Fig. 1) to obtain a large FEL gain. The FEL gain is close to the design value as confirmed from the satisfactory modulation in the spontaneous emission spectrum of the optical klystron ETLOK-III (Fig. 2). Further, to confine the spontaneous emission, highly stable optical resonators are placed at both ends of the infrared beam line (Fig. 3). In the optical resonators, the 3-axis translation and the 2-axis rotation are controlled independently, and the positional accuracy is 0.1 µm in the direction of the optical axis. We have a mechanism of suppressing any vibration from outside; the vibration displacement of the resonator mirror system is less than 0.5 µm.
Fig. 2 An example of spontaneous emission from the optical klystron ETLOK-III
The fundamental wavelength is in the infrared region and is invisible, but the third and fourth harmonics are in the visible region.
|
|
Fig. 3 Highly stable optical resonator
Two resonators are placed at both ends of the infrared beam line.
|
Adopting the above configuration, we succeeded for the first time in the world in the oscillation of a FEL using an infrared storage ring FEL system (Fig. 4). The oscillation wavelength ranges from 0.84 to 1.50 µm, and the maximum power is 1.6 mW. The maximum power suggests that a power of approximately 5 W is accumulated in the optical resonator. The wavelength is so stable and the relative line-width of a FEL beam is only less than 3x10-4, which is extremely small for a FEL in this wavelength region. The maximum brightness of the optical source is approximately 1015 photons/s/mm2/mrad2/0.1%b.w., which is considered as the world's largest for a storage ring light source in the infrared region.
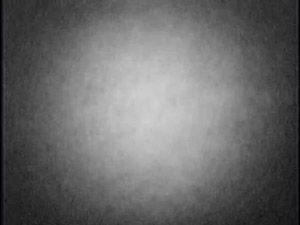 |
Fig. 4 Profile of an infrared FEL beam with the wavelength of 870 nm observed using a CCD camera
Picture size is approximately 4 mm (V) × 5.4 mm (H). The circular beam shows an almost complete Gaussian distribution, and the beam size agrees well with the value estimated from the wavelength. |
Up to 16 bunches of electrons can be stored in NIJI-IV, but the FEL oscillation needs only one electron bunch because the circumference of the storage ring is equal to the round length of the optical resonator. However, we need at least two bunches of electrons to emit X-rays using the inverse Compton scattering of FEL photons (Fig. 5). We can decide the locations for inverse Compton scattering of FEL photons by arbitrarily selecting two bunches, and therefore we have developed a system that can freely select them. With the selected two bunches of electrons, the infrared storage ring FEL was oscillated and a quasi-monochromatic X-ray was generated using the inverse Compton scattering of FEL photons (Fig. 6). By appropriately selecting the distance between electron bunches, we configured the collision point for inverse Compton scattering of FEL photons outside the magnetic field of the optical klystron, and an X-ray beam is emitted with an excellent monochromatic energy spectrum, as shown in Fig. 6.
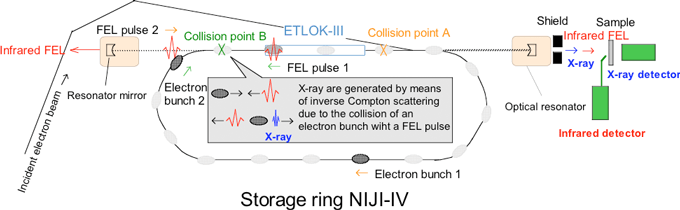 |
Fig. 5 Schematic view of inverse Compton scattering using an electron storage ring NIJI-IV
The light grey portions indicate where electron bunches can exist. Electron bunch 1 is synchronous with FEL pulse 1, and electron bunch 2 is synchronous with FEL pulse 2. Electron bunch 1 and FEL pulse 2 cause inverse Compton scattering at collision point A, and electron bunch 2 and FEL pulse 1 cause inverse Compton scattering at collision point B. If electron bunch 2 is in the grey section of medium density, it collides at that point with the FEL pulse 1. Therefore, we can decide the collision points by choosing the interval of electron bunches.
|
We are only second to Duke University in realizing the emission of high quality X-rays using inverse Compton scattering of FEL photons. We are the first to simultaneously emit these rays at multiple collision points using multiple FEL pulses. By controlling the wavelength of the FEL beam, we can vary the energy of quasi-monochromatic X-rays between 1.2 and 2.1 MeV, with the maximum flux of approximately 106 photons/s.
The quasi-monochromatic X-ray beam using the inverse Compton scattering of FEL photons has a pulse width of approximately 100 ps, which is 10 times larger than that of the FEL micropulse, and the X-ray beam is emitted synchronously in the same direction with the FEL beam. In other words, in the newly developed system, the infrared FEL beam from the storage ring and the quasi-monochromatic X-ray beam are emitted simultaneously. A possible application will be for the synchronous imaging with two frequency ranges and another will be for spectrometry in the infrared region, where we can obtain abundant information on molecular vibrations for detecting the changes in solid-state properties due to X-ray irradiation. The present study will pave the way for new research fields where infrared light and X-rays are used in combination.
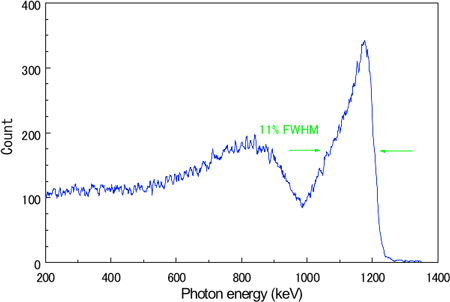 |
Fig. 6 A typical energy spectrum of X-rays generated by the inverse Compton scattering of FEL photons
In the figure, the full width at half maximum includes the contribution of the resolution of the detection system. The X-ray beam is quasi-monochromatic while the signal below 1 MeV is mostly due to the detector response.
|
The storage ring FEL system shall be upgraded to broaden the long wavelength side to reduce the energy of near monochromatic X-rays generated by the inverse Compton scattering. Further, the FEL technology with multi-bunch operation shall be developed to improve the X-ray beam intensity. Through these activities, we will prove that the inverse Compton scattering of FEL photons is a promising mechanism for realizing a quasi-monochromatic light source with variable energy down to 0.3 MeV.