- Opening the Way to Functional Devices in Combination with Organic Semiconductors -
The Correlated Electron Research Center (CERC), of the National Institute of Advanced Industrial Science and Technology (AIST), an independent administrative institution, has found a new method of developing organic ferroelectrics by use of acid-base type binary organic substances of π-electron system, in collaboration with the Japan Science and Technology Agency (JST), another independent administrative institution. The ferroelectricity in organic substances is a significant step for replacing the conventional inorganic materials for ferroelectric devices such as memory, and for exploiting emergent "gall-organic" devices. The present finding constitutes an important consequence for expanding the frontier of materials development.
Ferroelectric substances have a variety of functional device capabilities such as piezoelectric, actuator, non-linear optical devices, in addition to electronic applications such as non-volatile memory and high permittivity materials. For implementing these useful features in the form of light weight and flexible organic materials, needs for the organic ferroelectrics are growing steeply. On the other hand, ferroelectrics of low-molecular-weight organic solids are rather poor in the perfomance and also nearly untouched in the materials development, compared with ferroelectrics of inorganic or polymer.
A research team consisting of the CERC-AIST and the Tokura Spin Superstructure Project (Fiscal 2001~06), under the Exploratory Research for Advanced Technology Program (JST, ERATO-SSS) has demonstrated a ferroelectricity at near room temperature in molecular compounds prepared from non-polar conjugated molecules of binary acid-base by hydrogen-bonding. The development based on hydrogen-bonded molecular compounds is the first attempt in the world, and is expected to be useful for an efficient approach to ferroelectrics of low-molecular weight organic substances.
The newly developed ferroelectrics are characterized by large polarization and huge permittivity, as well as by electric field required for polarization reversal, much smaller than that of ferroelectric polymers. Besides, it has been found that the ferroelectric phase transition is a sort of barium titanate-type, attributable to relative displacement of nearly non-polar molecules.
Details of this finding will be published in the British Science Magazine "Nature Materials" February 1, 2005 issue (electronic edition: January 23, 2005). Title: "Ferroelectricity near room temperature in co-crystals of nonpolar organic molecules", by Sachio Horiuchi, Fumiyuki Ishii, Reiji Kumai, Yoichi Okimoto, Hiroaki Tachibana, Naoto Nagaosa, and Yoshinori Tokura.
A ferroelectric refers to a substance consisting of electric dipoles, which are spontaneously polarized, and of which polarity is readily reversed in response to applied electric field. Typical examples involve inorganic materials such as barium titanate, some lead-based oxides, sodium nitride, and so on (Fig. 1). Ferroelectric material has a broad variety of applications in electronics including capacitor, non-volatile memory, actuator and insulator for field-effect transistor (FET), and in other areas, such as non-linear optical device. Feasibility for additional applications and practical uses in varied fields is also suggested. In particular, its development has been actively pursued in view of the application to non-volatile memory element, FRAM or FeRAM, directly taking advantage of ferroelectric properties. For implementing such valuable functions with light weight and flexible materials, the development of organic ferroelectric is of great significance. On the other hand, poly(vinylidene fluoride) is well known as typical organic ferroelectric of solid state, except for the ferroelectric liquid crystal. In case of ferroelectric polymer, the reversal of polarization takes place when the substituents turn around the main chain structure in response to applied electric field. In the application to memory, high operating voltage is required for the reversal, disadvantageously. In order to obtain organic ferroelectrics of better performance, it is urgently desired to exploit organic molecules including those of low-molecular-weight, and to establish the strategy for materials development. Ferroelectricity in the low-molecular-weight organic solids have been known only a few cases, such as thiourea. It is no exaggeration to say that the materials development in this area is almost left untouched.
The available organic ferroelectrics of the low-molecular-weight substances are divided into two categories: single component substances and multi-component molecular compounds. In the former, the ferroelectricity is driven by the orientational reversal of the asymmetric polar molecules under electric field. The design principle of this type is based on the concept of order-disorder transition, which is exemplified by the ferroelectricity of sodium nitride having the permanent dipoles of NO2- ions. The permittivity of single component material at around the room temperature is about 30 for thiourea, and less than 15 for other substances. The molecular compound type involves charge transfer complex consisting of electron donor and acceptor. Polarization in charge transfer complex is caused by molecular displacement with electron transfer between molecules, and the maximum permittivity ranges from hundreds to around 2000 or greater. However, as the conductivity or dielectric loss is large, the material is not suited for insulating ferroelectric at present, in view of its own application target. In the present study, molecular compounds with strong hydrogen bond between acid and base have been selected as ferroelectric materials based on π-electron configuration in place of charge transfer complex.
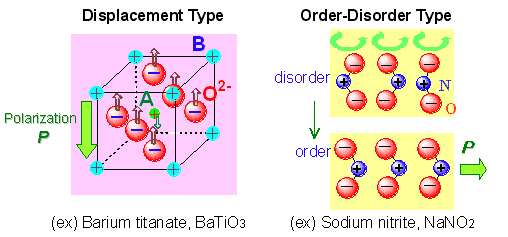 |
Fig. 1 Two types of ferroelectric substance |
The molecular compounds of 1:1 stoichiometry are created using dihydroxy-p-benzoquinone derivatives with strongly acidic (proton-donating) hydroxy groups, such as chrolanilic acid (H2ca) or bromanilic acid (H2ba) for acid, and phenazine (Phz) with proton accepting nitrogen atoms incorporated to a benzene ring for base (Fig. 2). Molecules of the two ingredients are of π-conjugated system, and one- dimensional network is formed through intermolecular hydrogen bonds. Fig. 3, left, shows the temperature dependence of permittivity measured on the single crystal specimen for the molecular compounds, Phz- H2ca and Phz- H2ba. While the transition temperature of Phz-H2ba is as low as 138 K, using smaller substituent can markedly improve the transition temperature, raising the transition point to 253 K for Phz-H2ca. Compressing Phz-H2ca at around 6000 atm makes phase transition occur at the room temperature. At the phase transition temperature, the permittivity grows steeply to implement a colossal value approaching to 3000 (Fig. 3, left). The permittivity at room temperature is greater than 100, far exceeding the values observed for the conventional organic ferroelectric substances.
At temperatures below the phase transition point, a polarization-electric field hysteresis curve is observed as shown in Fig. 3, right. With Phz-H2ca at 160 K, the remanent spontaneous polarization shown with Pr is relatively large at around 0.7~0.8 μC/cm2, while the electric field required for reversing the polarization, shown with Ec, is about 0.8 kV/cm, smaller than that of ferroelectric polymers by two to three orders of magnitude.
At temperatures higher than the phase transition point, both acid and base molecules are centrosymmetric without polarity. Below the transition point, their molecular centers are slightly displaced from the original centrosymmetric structure and deformation in the molecular skeletons is very small. This represents displacement (displacive-) type ferroelectricity like barium titanate. While the conventional displacement type ferroelectric produces polarization through the displacement of charged species (ions), in the newly developed ferroelectric, electrically neutral molecules are displaced, and the polarization of molecule itself remains very small. The future efforts will be concentrated in accounting for the origin of colossal spontaneous polarization observed.
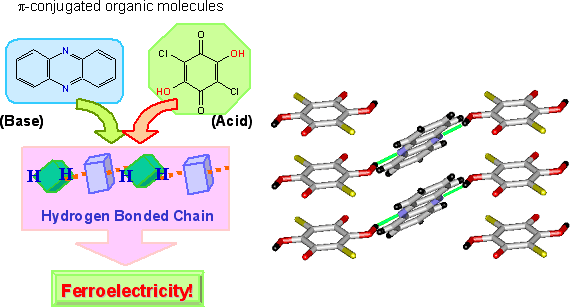 |
Fig. 2 Preparation of organic ferroelectric crystal based on π-conjugated molecules and its molecular arrangement (Green line: Hydrogen bonding).
|
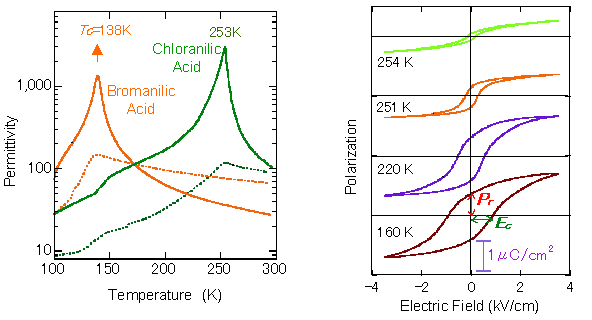 |
Fig. 3 Left: Temperature dependence of permittivity in ferroelectric crystals, phenazine-chloranilic acid and phenazine-bromanilic acid under ambient pressure (Solid and broken lines represent data with electric field applied parallel to and perpendicular to the molecular stack, respectively. Right: Polarization-electric field hysteresis curves of phenazine-chloranilic acid crystal, with electric field applied parallel to molecular stack.)
|